Basics of Nuclear Reactors
-
Nuclear reactors convert energy released by nuclear fission reaction to electricity
-
Control rods and containment structures help to safely regulate the energy-intensive process to avoid meltdowns
-
Designs of nuclear reactors over time have improved safety features, cost, and reactor lifetime
Overview of nuclear processes
Humans have developed ways to control the nuclear fission reaction (splitting the nucleus), releasing large amounts of nuclear energy in the form of heat. We harness the heat generated to generate electricity in nuclear reactors. For more on atomic and nuclear basics, see the nuclear fuels module.

How do nuclear reactors work?
The source of energy in a nuclear reactor is the nuclear fuel, often U-235 (an isotope or “type” of uranium). Once nuclear fission is initiated by bombardment with one neutron, released neutrons kick off a fission chain reaction that continues until a significant portion of the fuel is used up, or spent.

The tremendous amount of energy released from nuclear fission is used to heat a coolant, often water, which produces steam to turn a turbine to generate electricity. The coolant is then cooled back down and cycled back through the reactor chamber so it can be heated by the reaction again.

Figure modified and annotated from the US Department of Energy: Office of Nuclear Energy. This is typical for a Gen II nuclear reactor (see below).
Safety Considerations
Nuclear power plants require high initial capital expenditures in order to fully address safety concerns. The reactor chamber is located within a containment building, and various safeguards must be put in place to contain radioactive material and quickly cool down the energy-intensive process to avoid a meltdown.
The most common way to control nuclear reactions is to use a moderator, a material that absorbs neutrons and doesn’t release energy, slowing the overall chain reaction. The moderator is introduced in the form of control rods that are made of neutron-absorbing materials such as cadmium or hafnium. These control rods (which slow the nuclear reaction) alternate with fuel rods (the U-235 or other nuclear fuels where the chain reaction takes place) and can be raised or lowered to control the rate of the chain reaction.
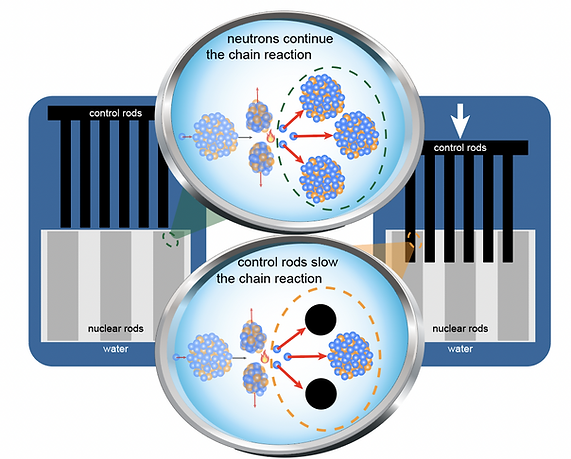
Nuclear reactors over time
Nuclear reactor technology is described in terms of generations. Some of the key considerations to balance when making new systems are cost, safety, security, grid accessibility, reactor lifetime, and the path to commercialization.
Gen I nuclear reactors were prototype reactors from the 1940s and 50s. However, when discussing nuclear reactors today, Gen II nuclear reactors are often the design that comes to mind. Gen II reactors were built in the late 1960s and designed with a 40-year lifetime. These mostly use water as a coolant, either in the form of pressurized water reactors (PWRs, see figure above) or boiling water reactors (BWRs), which refer to how the coolant water moves through the system.
Design improvements in the 1990s led to Gen III and Gen III+ reactors, which have the same basic technology as Gen II. Improvements include an increased lifetime (60 years), more efficient fuel use leading to less waste, and better safety measures. For more on radioactive waste, see Nuclear fuel fundamentals.
Gen II and III reactors are massive in size due to economies of scale: construction is time-consuming and expensive up front, so the historic approach has been to build big plants.
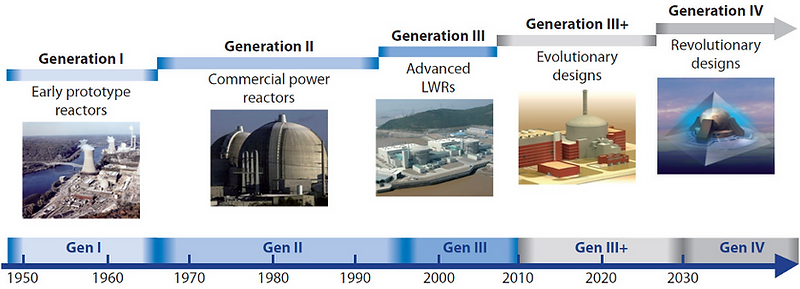
Timeline for different generations of nuclear reactors. Figure from Generation IV International Forum
New Technologies for Nuclear Reactors
Gen IV nuclear reactors are still in development and are receiving a lot of attention. The main focus of Gen IV systems is on developing small modular reactors (SMRs), which can be built more quickly and cheaply. The technological advances vary widely. Some designs are simply smaller versions of conventional reactors.
The most impactful new technological developments have focused on temperature and coolant. Reactors are being designed to operate at higher temperatures in order to be more efficient (which also decreases the chance of a meltdown because everything is already designed to withstand high temperatures). Instead of using water as a coolant, new systems are using coolants that can reach higher temperatures like molten salt, lead or sodium, or a gas such as helium. To improve efficiency, different parts of the reactor such as the reaction center and the generator can be combined. Finally, new safety features are being included in the design of these systems.
The safety improvements developed in new reactors generally rely on “passive” features that rely on gravity or natural convection. These are theoretically more fail-safe than mechanical pumps that rely on external energy sources or human control and are prone to failure.
Other focuses of research are working on developing microreactors, which are even smaller than Gen IV reactors and can work for 10 years without refueling. Ideally, the development of smaller systems could allow for deployment to different areas if safety and regulatory systems allow for this. Thorium reactors, which are based on thorium fuels rather than uranium, are also being developed.
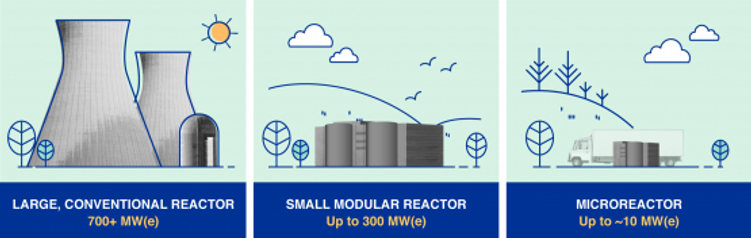
Learn more about
Questions for deeper thinking
-
Beyond waste generated, what impacts do nuclear reactors have on their surroundings, and how do new designs such as SMRs seek to address these challenges?
-
SMRs are still in development. Is it likely that they will ultimately be incorporated into our energy systems?
-
What are some benefits to developing microreactors?
Sources and further reading
-
Energy Information Agency (EIA): Nuclear Energy Explained
-
Energy Education: Nuclear Reactor
-
American Academy of Arts and Sciences: Nuclear Reactors
-
Energy Education: Generation IV Nuclear Reactors
-
Interesting Engineering: Micro Nuclear Reactors
-
US Department of Energy (DOE): How Does a Nuclear Reactor Work
Page last updated: August 31, 2022